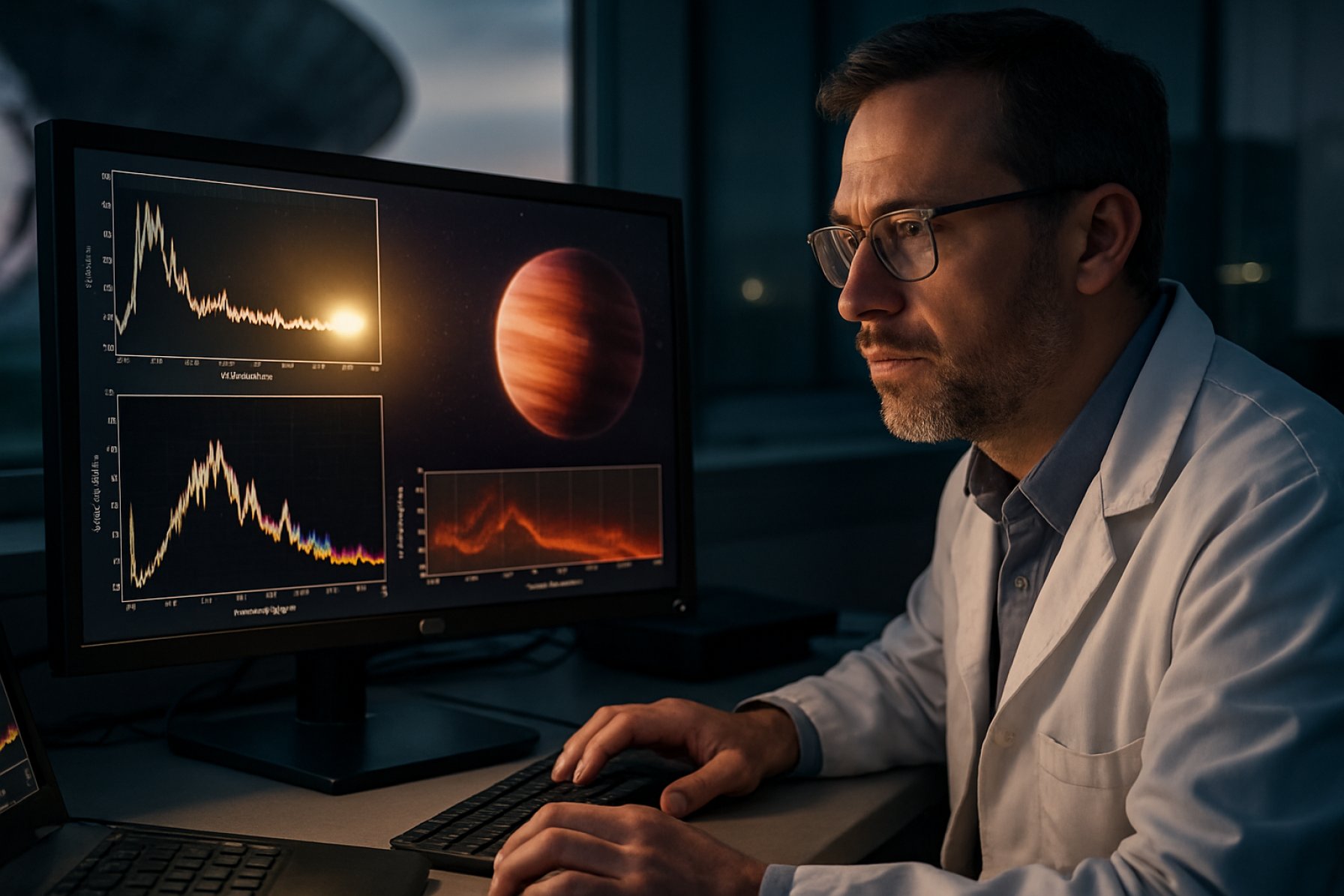
Table of Contents
- Executive Summary: The 2025 Flux Quantification Landscape
- Market Size & Growth Forecasts Through 2030
- Key Technological Advances in Exoplanetary Spectroscopy
- Leading Companies and Research Initiatives (e.g., nasa.gov, esa.int)
- Emerging Trends: AI, ML & Big Data in Flux Measurement
- Instrument Innovation: Next-Gen Spectrographs and Detectors
- Major Exoplanet Survey Missions and Collaborations
- Challenges: Calibration, Sensitivity, and Data Interpretation
- Investment Landscape and Funding Opportunities
- Future Outlook: Strategic Roadmaps and Disruptive Potential
- Sources & References
Executive Summary: The 2025 Flux Quantification Landscape
In 2025, flux quantification in exoplanetary spectroscopy stands at a pivotal juncture, driven by the deployment and maturation of advanced optical and infrared telescopes, as well as evolving analytical techniques. Accurately measuring the absolute and relative flux of exoplanetary systems—essential for inferring atmospheric composition, temperature profiles, and potential biosignatures—relies on a confluence of high-sensitivity instrumentation, robust calibration standards, and sophisticated data pipelines.
The successful operation of the National Aeronautics and Space Administration (NASA)’s James Webb Space Telescope (JWST) has already begun to reshape the flux quantification landscape. JWST’s Near Infrared Spectrograph (NIRSpec) and Mid-Infrared Instrument (MIRI) are delivering unprecedented sensitivity and wavelength coverage, enabling the detection of faint exoplanetary signals against stellar backgrounds. These capabilities have established new benchmarks for absolute flux calibration and noise floor characterization in exoplanetary transit and emission spectroscopy. In parallel, ongoing upgrades to the European Southern Observatory (ESO)’s Very Large Telescope (VLT) and the upcoming commissioning of the Extremely Large Telescope (ELT) are expected to further augment ground-based flux measurement precision through adaptive optics and next-generation spectrographs.
Data from 2024–2025 have highlighted the growing importance of cross-instrument calibration and standardized data reduction pipelines. Efforts such as the Infrared Processing and Analysis Center (IPAC)’s support for calibration reference standards and the ESO’s public data archives have accelerated inter-comparison and reproducibility across the community. Simultaneously, collaborative frameworks involving instrument manufacturers and research institutions are enabling the refinement of detector linearity, throughput models, and atmospheric correction algorithms. These developments are critical for translating raw detector counts into physical flux units with uncertainties below the percent level—a threshold necessary for robust atmospheric retrievals.
Looking ahead to the late 2020s, the deployment of missions such as European Space Agency (ESA)’s Ariel, focused exclusively on exoplanetary atmospheres, and the US-led Roman Space Telescope, will expand the observational parameter space, particularly in the mid-infrared and near-infrared regimes. Anticipated advances in detector technology, such as those pioneered by Teledyne Technologies Incorporated, are expected to further reduce readout noise and enhance dynamic range, directly benefiting flux quantification accuracy. Collectively, these initiatives position the field to transition from the era of detection to that of precise characterization, solidifying flux quantification as a linchpin of exoplanet science in 2025 and beyond.
Market Size & Growth Forecasts Through 2030
The market for flux quantification technologies in exoplanetary spectroscopy is poised for significant growth through 2030, driven by several converging factors within the astronomical instrumentation and advanced detector sectors. As of 2025, the deployment of next-generation space telescopes and ground-based observatories is rapidly expanding the addressable market for high-precision spectroscopic instruments and associated data analysis software. The launch of major missions such as the James Webb Space Telescope (JWST) and planned observatories like the Nancy Grace Roman Space Telescope by NASA and the European Space Agency’s Ariel exoplanet mission are creating substantial demand for flux quantification capabilities in both hardware and software domains.
Key players in the field, including Thales Group and Leonardo S.p.A., are actively supplying spectroscopic systems, detectors, and calibration sources vital for these missions. Meanwhile, established detector manufacturers such as Andover Corporation and Hamamatsu Photonics are innovating in the field of low-noise, high-sensitivity photodetectors tailored for faint flux measurements from distant exoplanets. Such advancements are directly expanding the commercial landscape for flux quantification equipment and services.
The market is further bolstered by the increasing volume of exoplanetary science programs funded by international agencies, including European Space Agency (ESA) and Japan Aerospace Exploration Agency (JAXA). The shift towards large, multi-institutional collaborations is driving demand for interoperable, scalable flux calibration and quantification solutions. This trend is especially prominent in the context of Extremely Large Telescopes (ELTs) such as those led by European Southern Observatory (ESO) and its partners, which require advanced flux measurement systems for their high-resolution instruments.
Through 2030, the market is expected to experience compounded annual growth as the cumulative number of exoplanet discoveries rises and as precision requirements for atmospheric characterization intensify. The emergence of dedicated software platforms and cloud-based flux quantification services from companies and institutions specializing in astronomical data processing is anticipated to further diversify the sector. With new missions and instrument upgrades continuously in the pipeline, the outlook remains robust, with flux quantification positioned as a foundational technology within the broader exoplanetary spectroscopy ecosystem.
Key Technological Advances in Exoplanetary Spectroscopy
Flux quantification stands as a cornerstone in exoplanetary spectroscopy, enabling the precise measurement of stellar and planetary light across a wide range of wavelengths. Recent years, particularly leading into 2025, have seen significant advances in both instrumentation and methodology, directly impacting the accuracy and reliability of flux measurements from distant worlds.
A major driver of progress is the deployment and ongoing operations of space-based observatories such as the National Aeronautics and Space Administration (NASA) James Webb Space Telescope (JWST). JWST’s Near-Infrared Spectrograph (NIRSpec) and Mid-Infrared Instrument (MIRI) offer unprecedented sensitivity and stability, allowing astronomers to detect and quantify flux variations from exoplanet atmospheres with high precision. Early science programs have already demonstrated the telescope’s ability to resolve subtle spectral features and absolute flux levels during transits and eclipses, a crucial step for characterizing atmospheric composition and thermal structures.
On the ground, new-generation spectrographs installed on extremely large telescopes (ELTs) are poised to further the field. Facilities like the European Southern Observatory’s Extremely Large Telescope (ELT), slated for first light by 2028, are developing high-resolution instruments equipped with stable calibration sources and advanced detectors. These systems are designed for superior flux calibration, reducing uncertainties caused by Earth’s atmosphere through real-time corrections and reference star monitoring. During 2025, commissioning activities for precursor instruments and adaptive optics systems are expected to deliver improved flux measurements from challenging targets, including those around faint or active stars.
Technological advances in detectors also play a pivotal role. Developments in mercury cadmium telluride (HgCdTe) infrared arrays and low-noise readout electronics, undertaken by industry leaders such as Teledyne Technologies Incorporated, are enhancing dynamic range and linearity in photon detection. These improvements facilitate more accurate flux quantification over a broad intensity range, which is essential for resolving both bright stellar and faint planetary signals in composite spectra.
Looking ahead, improved calibration pipelines and data reduction algorithms are expected to further boost flux accuracy. Open-source initiatives and cross-collaborations between space agencies and observatories are driving the standardization of flux calibration procedures—an essential step as multi-instrument and multi-epoch datasets become more common. The synergy between advanced hardware, robust software, and global collaborations is set to make flux quantification in exoplanetary spectroscopy both more precise and accessible in the years immediately beyond 2025.
Leading Companies and Research Initiatives (e.g., nasa.gov, esa.int)
Flux quantification in exoplanetary spectroscopy has emerged as a cornerstone of modern astrophysics, enabling researchers to characterize atmospheric compositions, thermal structures, and potential biosignatures on worlds beyond the solar system. The field is currently shaped by a combination of advanced observational platforms, pioneering research initiatives, and collaborative efforts among leading space agencies and institutions.
As of 2025, NASA continues to play a pivotal role through its flagship missions. The James Webb Space Telescope (JWST), launched in late 2021, remains at the forefront, delivering unprecedented spectroscopic data across the near- and mid-infrared. JWST’s cutting-edge instruments, such as NIRSpec and MIRI, allow for high-precision flux measurements, offering insights into exoplanetary atmospheres with a sensitivity far surpassing previous observatories. Through collaborative science teams and open-access data policies, NASA is fostering a global research ecosystem for the analysis of exoplanet flux and atmospheric retrieval models.
The European Space Agency (ESA) is also advancing the field with ongoing missions like CHEOPS and preparations for the Ariel mission, set for launch in 2029. Ariel, in particular, is designed to conduct a dedicated spectroscopic survey of hundreds of exoplanets, focusing on precise flux quantification across a broad wavelength range. In the run-up to Ariel’s launch, ESA is supporting international collaborations to refine calibration techniques and data analysis pipelines, ensuring robust flux measurements from its upcoming survey.
On the ground, the European Southern Observatory (ESO) is leveraging its suite of advanced telescopes, including the Very Large Telescope (VLT) and the forthcoming Extremely Large Telescope (ELT), both equipped with state-of-the-art spectrographs. These instruments are being optimized for high-resolution spectroscopy and accurate flux calibration, contributing critically to the study of transiting and directly imaged exoplanets.
In parallel, national observatories and academic consortia—such as those coordinated by JAXA and CNRS—are making strides in instrument development, data processing algorithms, and cross-validation of flux measurements. These collaborative networks are essential for standardizing methods and reconciling data across different platforms and observing strategies.
Looking ahead, the synergy between space-based and ground-based facilities, backed by the leadership of NASA, ESA, ESO, and other global partners, promises rapid advances in flux quantification techniques. These developments will underpin the next generation of exoplanetary atmospheric studies, paving the way for the systematic search for habitable environments and life beyond Earth.
Emerging Trends: AI, ML & Big Data in Flux Measurement
The integration of artificial intelligence (AI), machine learning (ML), and big data analytics is rapidly transforming the landscape of flux quantification in exoplanetary spectroscopy, especially as we enter 2025 and look ahead to the near future. These advanced computational approaches are addressing several critical challenges associated with analyzing vast, complex datasets generated by state-of-the-art space telescopes and ground-based observatories.
AI and ML algorithms are increasingly being employed to process and interpret the massive volumes of spectral data collected by next-generation instruments such as those aboard the National Aeronautics and Space Administration (NASA) and the European Space Agency (ESA). In particular, neural networks and deep learning models are now routinely used for de-noising spectra, correcting for instrument systematics, and extracting subtle exoplanetary signatures from stellar backgrounds. These capabilities are vital for robust flux quantification, especially when dealing with the low signal-to-noise ratios characteristic of exoplanetary transits and secondary eclipses.
Recent years have seen the implementation of ML-driven pipelines that automate much of the data reduction and flux calibration processes. For example, the ESA’s ARIEL mission, set for launch in the coming years, is actively developing machine learning frameworks to optimize the retrieval of atmospheric fluxes and molecular abundances from high-resolution spectra. Similarly, NASA’s James Webb Space Telescope (JWST), operational since 2022, has prompted a surge in AI-powered analysis tools designed to handle the unprecedented data throughput and complexity of its exoplanetary observations.
Big data infrastructure is also playing a critical role. Cloud-based platforms and distributed computing resources, often in collaboration with organizations like Amazon (through Amazon Web Services partnerships with public observatories), are enabling researchers to store, manage, and analyze petabyte-scale spectral archives efficiently. This, in turn, allows for more comprehensive meta-analyses and cross-mission studies, further enhancing the accuracy and reliability of flux quantification techniques.
Looking ahead, the synergy between AI, ML, and big data is expected to continue driving progress in exoplanetary flux measurement. Anticipated advancements include the deployment of self-improving, adaptive algorithms that can learn from new data in real time, as well as federated analytics that leverage distributed learning across diverse datasets and observatories. These trends will be instrumental in preparing for the next wave of missions—such as NASA’s Habitable Worlds Observatory—where the sheer scale and precision of flux measurements will demand unprecedented computational sophistication.
Instrument Innovation: Next-Gen Spectrographs and Detectors
Flux quantification in exoplanetary spectroscopy relies heavily on the continual advancement of spectrograph and detector technologies. As of 2025, the field is witnessing a marked acceleration in the deployment and refinement of next-generation instruments, both in ground-based observatories and through ambitious space missions. These innovations are directly addressing the persistent challenge of accurately measuring and interpreting the faint spectral fluxes from distant exoplanet atmospheres—often obscured by both instrumental noise and Earth’s atmospheric interference.
Key events include the ongoing commissioning and first science runs of instruments such as the Extremely Large Telescope’s (ELT) Mid-infrared ELT Imager and Spectrograph (METIS) and the High Resolution Spectrograph (HIRES). These spectrographs are designed with advanced adaptive optics and cryogenic detectors, enabling them to achieve unprecedented sensitivity and stability in the near- and mid-infrared regimes. The integration of large-format, low-noise HgCdTe and InSb arrays is central to improving photon collection efficiency and minimizing background noise, a critical factor in flux quantification for transiting and directly imaged exoplanets. The European Southern Observatory’s commitment to these projects signifies a major step forward in the field, with first-light science observations anticipated to begin in 2025 and ramp up in subsequent years (European Southern Observatory).
Simultaneously, the James Webb Space Telescope (JWST), operated by NASA, continues to deliver high-fidelity exoplanet spectra in both the near- and mid-infrared, with its NIRSpec and MIRI instruments setting new benchmarks for absolute flux calibration. The precise flux measurements from JWST are enabling the detection of subtle atmospheric features, such as water vapor, methane, and carbon dioxide, even in smaller and cooler exoplanets. The instrument teams are actively developing refined calibration pipelines and cross-instrument verification techniques to further reduce systematic uncertainties in flux measurement.
Looking ahead, the next few years will see the launch and commissioning of additional missions and instruments, such as the Atmospheric Remote-sensing Infrared Exoplanet Large-survey (ARIEL) by the European Space Agency, which will employ a dedicated array of spectrometers specifically optimized for flux quantification in a statistical sample of exoplanets. Detector manufacturers are also pushing the boundaries of sensitivity and linearity, with ongoing collaborations between scientific consortia and leading industry suppliers to produce custom sensor arrays tailored for ultra-precise flux measurements.
In summary, instrument innovation over 2025 and the following years is poised to transform flux quantification in exoplanetary spectroscopy, reducing systematic errors and enabling the characterization of ever-fainter and more complex planetary atmospheres.
Major Exoplanet Survey Missions and Collaborations
Flux quantification is central to exoplanetary spectroscopy, serving as the cornerstone for characterizing planetary atmospheres, compositions, and potential biosignatures. As of 2025, this field is experiencing rapid advancements, enabled by collaborative efforts among space agencies, research institutions, and private organizations. Major survey missions are driving improvements in sensitivity, calibration, and data analysis techniques, thereby enhancing the precision and reliability of flux measurements.
The National Aeronautics and Space Administration (NASA)‘s James Webb Space Telescope (JWST), launched in late 2021, continues to set new standards in exoplanetary flux quantification. JWST’s Near-Infrared Spectrograph (NIRSpec) and Mid-Infrared Instrument (MIRI) have delivered unprecedented spectra of exoplanet atmospheres, directly quantifying flux variations during planetary transits and eclipses. These data are enabling the retrieval of molecular abundances and thermal structures with high fidelity, fostering breakthroughs in our understanding of planet formation and habitability.
Meanwhile, the European Space Agency’s ESA ARIEL mission, scheduled for launch in 2029 but currently in advanced development and calibration phases, is poised to expand flux quantification capabilities through simultaneous multi-wavelength observations of hundreds of exoplanets. ESA’s ongoing CHEOPS and PLATO missions also contribute by providing high-precision photometry, crucial for accurate baseline flux measurements and transit depth estimations.
Ground-based observatories are further enhancing flux quantification. The European Southern Observatory (ESO) operates the Very Large Telescope (VLT), which utilizes instruments such as ESPRESSO and CRIRES+ to acquire high-resolution spectra and precise flux measurements. The upcoming Extremely Large Telescope (ELT), slated for first light in the late 2020s, promises to surpass current capabilities with a 39-meter aperture optimized for faint flux detection and detailed atmospheric characterization.
In parallel, collaborations with detector manufacturers and calibration standards providers, such as Teledyne Technologies (noted for their infrared detectors used in JWST and future missions), are refining the absolute flux calibration chain, reducing uncertainties, and enabling cross-instrument consistency.
Looking ahead, the next few years will see a convergence of space- and ground-based efforts, with data-sharing initiatives and open-source analysis tools accelerating progress in flux quantification. As missions like JWST accumulate long-term time series and new platforms come online, the exoplanet community expects to achieve unprecedented sensitivity to atmospheric signatures, directly informing the search for life beyond Earth.
Challenges: Calibration, Sensitivity, and Data Interpretation
Flux quantification in exoplanetary spectroscopy is pivotal for determining atmospheric composition, thermal structure, and potential biosignatures on distant worlds. However, the field faces notable challenges in calibration, sensitivity, and data interpretation, especially as observational capabilities expand in 2025 and the near future.
Calibration remains a cornerstone challenge. Accurate flux measurements require rigorous instrument calibration to correct for detector response, optical throughput, and time-dependent systematics. Space-based observatories such as the National Aeronautics and Space Administration (NASA)’s James Webb Space Telescope (JWST) currently set the benchmark for calibration protocols, employing frequent reference observations and onboard calibration sources. Nevertheless, even with JWST’s advanced systems, residual uncertainties from pointing jitter, detector non-linearity, and thermal variations persist. The upcoming European Space Agency (ESA) ARIEL mission, scheduled for launch in 2029, is actively developing new calibration schemes tailored to high-precision flux retrieval across a wide spectral range, with early testbeds and ground-based prototypes already in operation as of 2025.
Sensitivity is equally crucial due to the extremely faint signals from exoplanet atmospheres. The photon noise limit, background emission, and detector dark currents all constrain achievable sensitivity. Recent advancements in mid-infrared detector technology, such as those manufactured by Teledyne Technologies Incorporated, have improved array uniformity and noise performance, aiding the extraction of weak exoplanetary fluxes. However, as astronomers push toward characterizing smaller, cooler exoplanets, the demand for even higher sensitivity and lower noise floors will intensify, especially for ground-based instruments battling atmospheric absorption and emission.
Data Interpretation introduces further complexity. Flux measurements must be disentangled from stellar activity, instrumental systematics, and interstellar absorption, all of which can masquerade as or obscure genuine planetary signals. The interpretation pipeline relies on sophisticated radiative transfer models and robust statistical methods to separate planetary flux from confounding sources. Collaborations between agencies like NASA, ESA, and detector suppliers are driving the refinement of these models, but the influx of high-resolution, high-cadence data expected in coming years will require substantial upgrades to both computational tools and community-wide data standards.
Looking ahead, coordinated calibration campaigns, continued detector innovation, and open-source data analysis platforms are expected to underpin progress in flux quantification. The anticipated synergy between JWST, ARIEL, and ground-based observatories such as those operated by the European Southern Observatory is likely to yield unprecedented constraints on exoplanet atmospheres, provided these persistent challenges are met with sustained technical and methodological advances.
Investment Landscape and Funding Opportunities
The investment landscape for flux quantification in exoplanetary spectroscopy is experiencing robust growth as both public and private sectors recognize the scientific and commercial potential of advanced astronomical instrumentation. Major investments are being channeled into the development of next-generation space telescopes and ground-based observatories, alongside the refinement of photometric and spectroscopic analysis tools essential for precise flux measurement of exoplanetary atmospheres. The year 2025 marks a pivotal period, with several flagship projects reaching critical milestones and new funding initiatives emerging.
Governmental space agencies remain principal drivers of funding and technology development in this arena. NASA’s ongoing support for missions such as the James Webb Space Telescope (JWST) and the Nancy Grace Roman Space Telescope underscores a sustained commitment to exoplanet science and, specifically, to improving the accuracy of flux calibration in spectroscopic observations. In 2025, NASA-backed programs are allocating resources to enhance detector sensitivity and calibration standards, thereby enabling more precise quantification of stellar and planetary fluxes.
International collaboration is also intensifying. European Space Agency (ESA) is advancing its PLATO and ARIEL missions, both of which prioritize high-precision photometric and spectroscopic measurements. These missions have drawn funding from both pan-European research initiatives and member state contributions. ESA’s collaborations with national research councils and universities are facilitating technology transfer and training, broadening the investment base beyond traditional aerospace entities.
On the commercial side, private aerospace firms are increasingly active in the development of advanced detectors, spectrometers, and calibration sources. Companies such as Thales Group and Teledyne Technologies are receiving contracts and venture investment to design and manufacture high-efficiency infrared detectors and precision optics tailored for exoplanetary research. These firms benefit from public-private partnerships, often leveraging government grants to de-risk early-stage technology development.
Looking ahead, the next few years are expected to see new funding calls from organizations like National Science Foundation (NSF) for instrument development and data analysis tools that enhance flux quantification capabilities. Venture capital and philanthropic foundations are also showing interest, particularly as the potential for interdisciplinary applications—such as climate modeling and remote sensing—becomes apparent. Overall, the funding environment in 2025 and beyond is characterized by a convergence of public investment, international cooperation, and growing private sector participation, all driving advancements in exoplanetary flux quantification technologies.
Future Outlook: Strategic Roadmaps and Disruptive Potential
Flux quantification stands as a cornerstone for advancing exoplanetary spectroscopy, directly influencing our ability to characterize distant worlds. The strategic trajectory for the field in 2025 and the near future is defined by the convergence of increasingly sensitive instrumentation, advanced calibration protocols, and the integration of novel computational methods.
The impending launch and early operations of the European Space Agency‘s Ariel mission, scheduled for 2029, are already shaping industry priorities. Ariel’s dedicated approach to spectroscopically surveying hundreds of exoplanetary atmospheres will require unprecedented flux calibration strategies, pushing suppliers and instrument designers to refine detector linearity, absolute photometric accuracy, and in-orbit calibration methods. Industry partners such as Airbus are collaborating on payload and platform development, emphasizing robust flux stability to ensure the reliability of derived atmospheric spectra.
Meanwhile, ground-based platforms are entering a phase of rapid enhancement, with major observatories deploying or upgrading high-resolution spectrographs. The European Southern Observatory is at the forefront, with its Extremely Large Telescope (ELT) expected to come online in 2028. The ELT’s spectrographs, including METIS and HIRES, are being designed for ultra-precise flux measurements across infrared and optical bands, demanding stringent calibration standards and new flux referencing protocols.
Flux quantification is also benefiting from disruptive advances in detector technology. Companies such as Teledyne Technologies are supplying next-generation infrared detectors with improved quantum efficiency and lower noise, enabling more accurate photon counting in faint exoplanet signals. These advances are critical as researchers seek to move from mere detection to quantifiable characterization of molecular abundances and energy budgets in exoplanetary atmospheres.
On the computational front, the adoption of machine learning and Bayesian inference methods is reshaping how flux uncertainties are modeled and propagated through atmospheric retrieval pipelines. Collaborative initiatives between observatories and computation-focused companies are expected to mature further by the late 2020s, streamlining the extraction of reliable fluxes from complex, noise-dominated datasets.
Looking ahead, the sector anticipates that the synergy between high-stability instrumentation, real-time calibration, and data-driven analysis will enable the next leap in exoplanetary science. Strategic roadmaps from space agencies and major industrial partners are increasingly aligned: precise flux quantification will be pivotal not only for fundamental discoveries but also for informing the habitability assessment of Earth-like worlds in the coming decade.
Sources & References
- National Aeronautics and Space Administration (NASA)
- European Southern Observatory (ESO)
- Infrared Processing and Analysis Center (IPAC)
- European Space Agency (ESA)
- Teledyne Technologies Incorporated
- Thales Group
- Leonardo S.p.A.
- Andover Corporation
- Hamamatsu Photonics
- Japan Aerospace Exploration Agency (JAXA)
- CNRS
- National Aeronautics and Space Administration
- European Space Agency
- Amazon
- National Science Foundation
- Airbus
- European Southern Observatory
- Teledyne Technologies