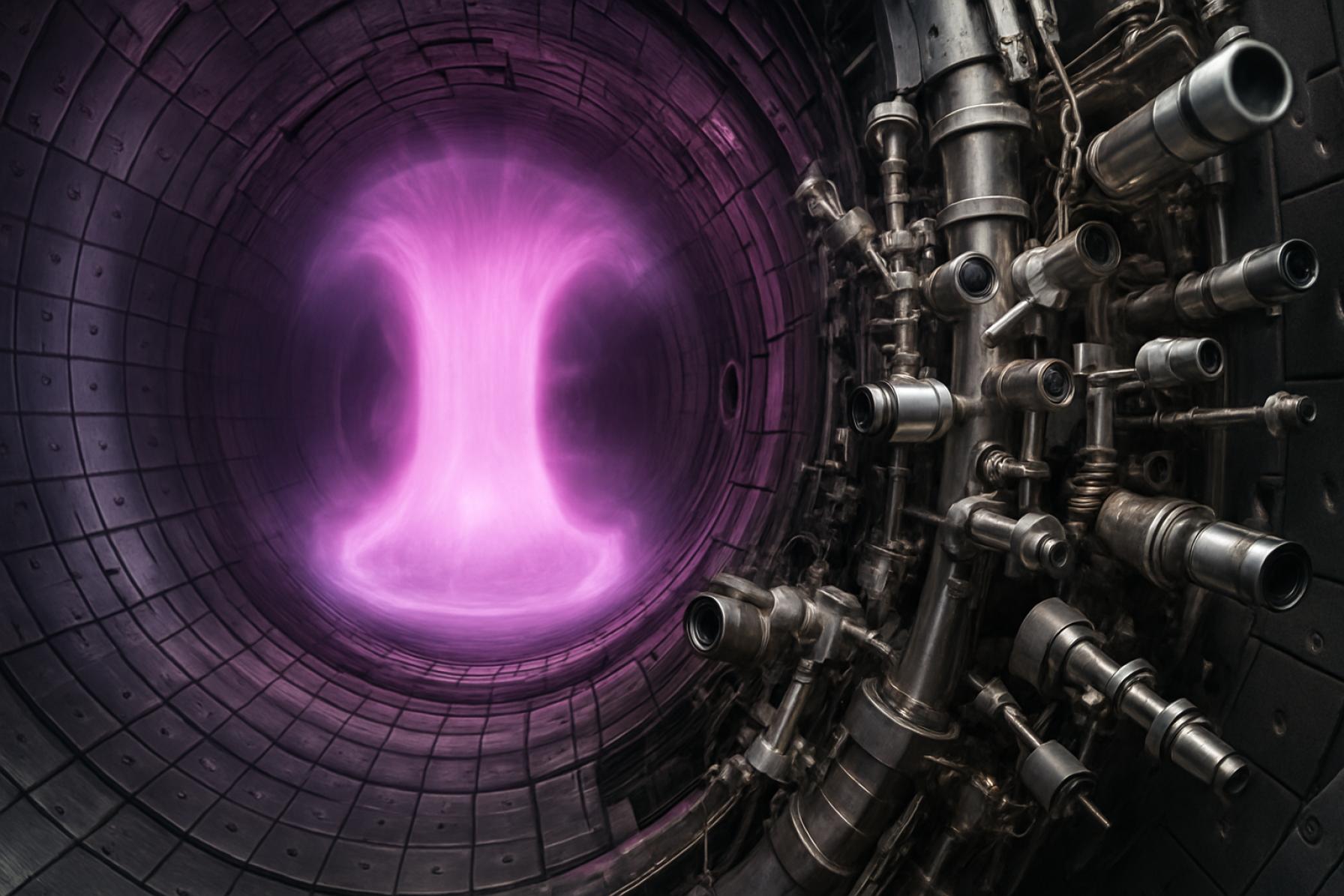
Table of Contents
- Executive Summary: Fusion Power’s Diagnostic Revolution
- Market Size & Growth Forecasts for 2025–2029
- Key Players: Leading Companies and Emerging Innovators
- Core Technologies in Tokamak Plasma Diagnostics
- Recent Breakthroughs and Cutting-Edge Research
- Challenges: Engineering, Cost, and Data Analysis Hurdles
- Global Collaboration: Major Projects and Industry Alliances
- Applications Beyond Fusion: Broader Impacts of Diagnostics
- Investment Trends and Funding Opportunities
- Future Outlook: Roadmap to Commercial Fusion Energy
- Sources & References
Executive Summary: Fusion Power’s Diagnostic Revolution
Tokamak plasma diagnostics engineering is experiencing a pivotal transformation as fusion research accelerates worldwide. In 2025, major milestones are being achieved in the development, deployment, and integration of advanced diagnostics systems designed to support next-generation fusion reactors. These systems are crucial for real-time measurement and control of plasma parameters such as temperature, density, impurity content, and magnetic field configurations, all of which are vital to sustaining stable plasma and achieving net energy gain.
Leading international fusion projects, including ITER Organization and EUROfusion, have advanced the engineering of diagnostics suites, focusing on robustness, spatial/temporal resolution, and resilience to harsh neutron and gamma environments. ITER’s diagnostics program alone involves over 45 major systems, with significant progress in 2024-2025 on the integration of key sensors such as bolometers, Thomson scattering systems, and neutron flux monitors. The deployment of these systems marks the first large-scale industrialization of fusion diagnostics, setting new standards for component reliability and data acquisition rates.
Meanwhile, collaborations with industry leaders like Thales and Mirion Technologies are driving the development of radiation-hardened detectors, high-speed data links, and advanced signal-processing electronics. These partnerships are enabling diagnostics platforms that can deliver real-time, actionable insights for plasma control and machine protection, a critical requirement as devices like UK Atomic Energy Authority’s MAST Upgrade and ITER approach deuterium-tritium operation phases.
Commercial fusion startups—including Tokamak Energy and TAE Technologies—are also investing heavily in proprietary diagnostic solutions tailored to their unique tokamak and alternative confinement configurations. These efforts emphasize compact, modular diagnostics designed for rapid deployment and remote monitoring, reflecting the industry’s shift toward scalable, reproducible fusion plant designs.
Looking ahead to the late 2020s, the outlook for tokamak plasma diagnostics engineering is defined by ongoing digitalization, AI-driven data analysis, and expanded use of remote and autonomous systems. The fusion sector’s embrace of advanced diagnostics is expected to accelerate progress toward demonstration reactors and, ultimately, commercial fusion power plants, with standards and best practices increasingly codified through international collaborations.
Market Size & Growth Forecasts for 2025–2029
The market for Tokamak Plasma Diagnostics Engineering is anticipated to experience robust growth from 2025 through 2029, driven by increasing investments in fusion research and the ongoing construction and operation of large-scale experimental facilities worldwide. The global focus on advancing fusion as a sustainable energy source is stimulating demand for sophisticated diagnostic systems capable of providing real-time, high-resolution measurements of plasma parameters within tokamaks.
A key driver of market expansion is the progress of flagship projects such as the International Thermonuclear Experimental Reactor (ITER), which is entering advanced operational phases through 2025 and beyond. ITER’s procurement and integration of over 50 distinct plasma diagnostic systems—ranging from magnetic sensors and Thomson scattering to neutron and impurity monitors—represent significant capital expenditure and engineering demand in the sector (ITER Organization). Similarly, the forthcoming commissioning of the China Fusion Engineering Test Reactor (CFETR), scheduled for late this decade, is expected to further boost global requirements for high-precision diagnostics (Institute of Plasma Physics, Chinese Academy of Sciences).
On the supplier side, companies specializing in diagnostic hardware—such as D-TACQ Solutions Ltd (fast data acquisition systems), HiTec Zang (customized plasma measurement solutions), and American Superconductor Corporation (magnetic sensors)—are expanding their product lines and R&D investments to meet project-specific requirements. The market is also witnessing increased collaboration between manufacturers and research consortia to develop next-generation diagnostics for extreme plasma environments.
- Market Value: While specific revenue figures for the global tokamak diagnostics market are typically proprietary, estimates from sector-involved organizations suggest compound annual growth rates (CAGR) in the range of 8–12% through 2029, with the total market value projected to reach several hundred million USD by the end of the forecast period. This trajectory is underpinned by both the retrofitting of existing tokamaks and deployment in new experimental machines.
- Regional Outlook: Europe, China, Japan, and the United States continue to be the leading markets, driven by projects like ITER, CFETR, JT-60SA, and the DIII-D National Fusion Facility (ITER Organization, Japan Atomic Energy Agency, General Atomics).
- Growth Catalysts: Increased public funding for fusion R&D, private sector interest in commercial fusion pilot plants, and the need for advanced diagnostic capabilities as plasma performance thresholds rise.
Looking forward, the market is poised for steady expansion, with innovation in real-time data processing, machine learning integration, and radiation-hardened components identified as emerging trends likely to shape the competitive landscape for tokamak plasma diagnostics engineering through 2029.
Key Players: Leading Companies and Emerging Innovators
The landscape of tokamak plasma diagnostics engineering in 2025 is distinguished by a combination of established industrial leaders and agile emerging innovators. These organizations are instrumental in advancing the precision, reliability, and integration of diagnostic systems—an essential component for successful plasma control and, ultimately, fusion energy realization.
- ITER Organization: As the world’s largest fusion experiment, ITER is a focal point for diagnostic engineering. Their extensive suite of over 50 diagnostic systems—ranging from neutron and gamma detectors to sophisticated optical and magnetic probes—are under final stages of deployment and commissioning, with first plasma operations slated for late 2025. ITER’s diagnostic procurement involves major industrial collaborations across Europe, Japan, India, the US, and South Korea, with continuous updates on component delivery and installation milestones provided by the ITER Organization.
- Tokamak Energy Ltd: This UK-based private company is pushing compact spherical tokamak designs and corresponding diagnostic technologies. Tokamak Energy’s ST40 device, for example, is equipped with advanced Thomson scattering, fast camera imaging, and multi-channel magnetic diagnostics, with ongoing R&D in real-time data acquisition and AI-driven analysis platforms. Details of these advancements are regularly reported by Tokamak Energy Ltd.
- General Atomics: Operating the DIII-D National Fusion Facility, General Atomics is a pioneer in developing and deploying new plasma diagnostic techniques such as charge exchange recombination spectroscopy, advanced bolometry, and electron cyclotron emission diagnostics. Their engineering teams are focused on upgrading systems for higher resolution and integration with machine learning feedback, as highlighted by General Atomics.
- Mirion Technologies: Specializing in radiation measurement, Mirion supplies neutron and gamma diagnostics for fusion applications, including tailored sensors and data electronics compatible with harsh tokamak environments. Their products are integrated into both ITER and national fusion experiments, as documented by Mirion Technologies.
- AMETEK (Princeton Applied Research): Providing precision electronic instrumentation, AMETEK develops diagnostic tools for plasma parameter measurement and real-time control, supporting global tokamak projects as described on the AMETEK corporate site.
- Emerging Innovators: Start-ups such as Commonwealth Fusion Systems and Helion Energy are designing next-generation diagnostics tailored for compact, high-field devices. Their focus is on modular, robust diagnostic modules and leveraging AI-driven data pipelines to enable faster experimental turnarounds.
As fusion research accelerates toward demonstration power plants, collaboration between these key players and a growing ecosystem of suppliers and integrators is expected to yield significant advances in diagnostic capabilities, supporting both experimental insight and the eventual transition to commercial fusion energy.
Core Technologies in Tokamak Plasma Diagnostics
Tokamak plasma diagnostics engineering is at the forefront of enabling controlled fusion by providing real-time, high-precision measurements of plasma properties critical for reactor stability and performance. In 2025 and the near future, advancements in core diagnostic technologies are closely tied to the escalating needs of large-scale projects like ITER, as well as the rapid progress of private-sector fusion ventures.
A cornerstone technology remains Thomson scattering systems, which offer localized, non-perturbative measurements of electron temperature and density. ITER’s multi-laser Thomson scattering diagnostic is slated for initial deployment in 2025, pushing the limits of spatial and temporal resolution over a wide plasma region. The engineering challenges associated with integrating these systems—such as maintaining optical alignment, mitigating neutron-induced damage, and automating calibration—are driving innovations in optics and materials, with leading contributions from Tokamak Energy and ITER Organization.
Magnetic diagnostics, including Mirnov coils and Rogowski loops, are being refined to withstand harsh fusion environments and provide higher bandwidth measurements. The next generation of magnetic sensors employs ceramic insulators and fiber-optic technologies to ensure resilience to radiation and electromagnetic noise. Companies like Kyocera are supplying advanced ceramics for sensor encapsulation, while Thorlabs is supporting optical transmission components.
Bolometry and soft X-ray diagnostics are evolving with the integration of semiconductor-based detectors, improving spatial resolution and allowing tomographic reconstruction of radiation profiles. Canon and Hamamatsu Photonics are at the forefront of supplying high-sensitivity photodiodes and camera systems for these applications. These detectors are being tailored to survive ITER’s neutron flux and are seeing deployment in pilot plants operated by private fusion companies.
Another area of active development is the use of millimeter-wave and microwave diagnostics, such as reflectometry and electron cyclotron emission (ECE) systems, for real-time plasma control. TOPTICA Photonics and Radiometer Physics GmbH are partnering with fusion research labs to deliver frequency-agile sources and receivers capable of withstanding the high-radiation, high-temperature environments inside next-generation tokamaks.
Looking ahead, the fusion community is investing in robust, automated diagnostic suites with integrated machine learning for anomaly detection and predictive maintenance. These systems are expected to be standard in demonstration plants by the late 2020s, as exemplified by the diagnostic integration efforts underway at ITER Organization and Tokamak Energy.
Recent Breakthroughs and Cutting-Edge Research
Tokamak plasma diagnostics engineering is experiencing rapid advancements as the global fusion community intensifies efforts towards achieving practical fusion energy. In 2025, significant breakthroughs are emerging, particularly in the integration of advanced sensors, real-time data analytics, and robust engineering solutions for next-generation tokamak devices.
One of the most notable developments is the deployment of comprehensive diagnostic suites in large-scale international projects such as ITER. The ITER diagnostic system, comprising over 50 individual diagnostic technologies, is nearing completion and entering its commissioning phase. These diagnostics include magnetic sensors, bolometry, neutron detection, Thomson scattering, and advanced spectroscopic systems, all engineered for long-term operation under intense neutron and thermal loads. The ITER Organization reports ongoing installation and validation of these systems, setting new standards in reliability and data integration for future reactors.
In parallel, research tokamaks like the Joint European Torus (JET) and Korea Superconducting Tokamak Advanced Research (KSTAR) are pioneering upgrades in diagnostic hardware and software. The EUROfusion consortium has overseen the implementation of high-resolution fast cameras and improved laser-based diagnostics at JET, enabling unprecedented visualization of plasma instabilities and impurity transport. Similarly, the National Fusion Research Institute in Korea has advanced the use of multi-dimensional imaging diagnostics, contributing to enhanced plasma control capabilities at KSTAR.
- Machine Learning Integration: In 2025, real-time machine learning algorithms are being embedded into diagnostic systems to handle the immense data streams generated by modern tokamaks. These AI-driven solutions, supported by organizations such as ITER Organization and EUROfusion, are enabling automated event detection, anomaly identification, and predictive maintenance—critical for safe and efficient reactor operation.
- Radiation-Hardened Engineering: Diagnostic components are now routinely designed with advanced materials and shielding techniques to withstand high neutron fluence, as documented by ITER Organization. These engineering solutions extend the operational lifespan of sensors and electronics, reducing maintenance downtime and costs.
- Outlook: As fusion projects transition toward steady-state operation (notably at ITER and the upcoming Chinese CFETR), the demand for robust, high-fidelity diagnostics is expected to grow. Companies specializing in sensor technology and system integration, such as American Superconductor Corporation (AMSC) and Tokamak Energy, are investing in new generations of diagnostic tools tailored for commercial reactor environments.
Overall, the period from 2025 onward is set to witness the maturation of tokamak plasma diagnostics engineering, with a strong emphasis on data integration, operational resilience, and real-time control, all of which are essential for the realization of sustainable fusion power.
Challenges: Engineering, Cost, and Data Analysis Hurdles
The engineering of plasma diagnostics for tokamaks faces a confluence of technical, economic, and analytical challenges that continue to shape progress in 2025 and are expected to remain central in the coming years. The complexity of acquiring reliable, high-fidelity data from the harsh environment inside a tokamak is compounded by the need for robust components, high temporal and spatial resolution, and the integration of advanced data analysis tools.
From an engineering perspective, diagnostics systems must withstand intense neutron and gamma radiation, high heat fluxes, and strong electromagnetic interference. This necessitates the use of specialized materials and shielding, as well as remote handling capabilities for maintenance and upgrades. At ITER Organization, current efforts focus on qualifying diagnostic components that can survive up to 20 years of operation under such extreme conditions, including the development of radiation-hardened sensors and optical systems. Issues such as maintaining calibration, reliability, and access for repairs remain significant engineering hurdles, especially as machines scale toward reactor-relevant conditions in new tokamaks like EUROfusion’s DEMO.
Cost is another considerable barrier. The diagnostic suite for a modern tokamak can represent as much as 15% of the total device budget, due to the high degree of customization, strict regulatory requirements, and the need for continuous R&D. For example, ITER’s diagnostic systems have an estimated cost exceeding €600 million, reflecting not only the hardware but also the complexity of integration and commissioning (ITER Organization). Smaller commercial ventures, such as Tokamak Energy and TAE Technologies, are investing in more modular and cost-effective diagnostic solutions to lower barriers for future commercial reactors.
On the data analysis front, the sheer volume and complexity of output from multi-modal diagnostics present ongoing challenges. Real-time interpretation of signals from hundreds of sensors, sometimes generating petabytes of data per experiment, requires advanced algorithms and high-performance computing resources. The fusion community is increasingly leveraging machine learning and artificial intelligence to automate event detection, anomaly identification, and predictive maintenance scheduling (ITER Organization). However, the scarcity of expertly labeled datasets and the need for cross-device validation remain technical bottlenecks.
Looking ahead, overcoming these hurdles will require collaborative engineering, standardization of interfaces, and continued investment in both hardware and digital infrastructure. The next few years are likely to see incremental improvements in diagnostic resilience, cost efficiency, and data processing capabilities, all of which are essential for the reliable operation and scaling of future fusion power plants.
Global Collaboration: Major Projects and Industry Alliances
Tokamak plasma diagnostics engineering stands at the confluence of advanced instrumentation and international scientific collaboration, a dynamic notably visible in major projects and industry alliances shaping the field through 2025 and beyond. The scale and complexity of modern tokamaks, such as ITER and the China Fusion Engineering Test Reactor (CFETR), demand sophisticated diagnostics systems and unprecedented coordination among global partners.
At the heart of these efforts is ITER Organization, whose diagnostic systems—ranging from magnetic sensors to Thomson scattering and neutron detection—have required the involvement of leading engineering firms and national laboratories from across the ITER member states. By 2025, ITER’s diagnostics installation is advancing in tandem with the tokamak’s assembly, with several prototyped subsystems entering the final integration phase. The European Domestic Agency (Fusion for Energy) is responsible for key systems such as the bolometric and visible/infrared diagnostics, while India and Russia supply crucial components like the electron cyclotron emission and soft X-ray diagnostics, respectively.
Meanwhile, China’s Institute of Plasma Physics, Chinese Academy of Sciences (ASIPP) is pushing forward with diagnostics for CFETR, focusing on high-resolution systems for edge plasma and core fluctuation measurements. These efforts are underpinned by partnerships with domestic manufacturers and global companies, leveraging expertise in optical and electromagnetic diagnostics. ASIPP’s collaborations, including with Hefei Bochuang Power Technology Co., Ltd. and international suppliers, are targeting improved diagnostic resilience against harsh neutron and gamma environments, a key requirement for steady-state fusion operation.
On the industry front, companies such as Thermo Fisher Scientific and Oxford Instruments are supplying advanced detectors, amplifiers, and magnetic sensing technology to research consortia. These collaborations facilitate technology transfer, drive miniaturization, and enhance signal processing capabilities, directly supporting the diagnostic needs of both government-led and emerging private fusion projects.
Outlook for the next few years centers on continued convergence of public and private sectors. International working groups coordinated by the International Atomic Energy Agency (IAEA) are establishing standards for diagnostic data interoperability and radiation hardness. As next-generation tokamaks such as JT-60SA (Japan) and SPARC (USA) advance, shared engineering solutions and cross-border supplier networks are expected to further accelerate innovation and reduce costs, reinforcing the global nature of tokamak plasma diagnostics engineering.
Applications Beyond Fusion: Broader Impacts of Diagnostics
Tokamak plasma diagnostics engineering, traditionally central to controlled fusion research, is increasingly finding impactful applications beyond its original domain. As the sophistication of diagnostic tools and analysis methods advances—driven by the needs of projects like ITER Organization and the forthcoming EUROfusion DEMO reactor—these technologies are being adapted for use across multiple scientific and industrial sectors.
High-resolution imaging systems, such as those developed for real-time monitoring of plasma instabilities, are being deployed in materials science for the study of rapid phase transitions and stress responses in advanced alloys. For example, fast bolometers and X-ray tomography systems, originally designed for tokamak experiments, are now enabling non-destructive testing (NDT) and dynamic failure analysis in aerospace and automotive manufacturing. Companies like Mirion Technologies, a supplier to fusion projects, have begun offering adapted radiation and imaging detection systems for industrial quality assurance and safety monitoring.
Another significant crossover is in medical diagnostics. Advanced spectroscopic techniques for measuring impurity content and plasma composition—refined by tokamak engineers—are being applied in next-generation medical imaging and cancer therapy. Oxford Instruments, a major supplier of magnetic measurement technology for fusion research, now markets their high-field magnetometers and sensors for use in MRI systems and precision radiotherapy.
Environmental monitoring also benefits from these diagnostic advances. Laser-based interferometry and Thomson scattering, standard in plasma diagnostics, are being utilized for real-time atmospheric pollution measurement and gas analysis. Thorlabs, a provider of photonics equipment to fusion laboratories, has expanded its product lines to support environmental sensing and air quality monitoring initiatives worldwide.
Looking to 2025 and beyond, key fusion diagnostic suppliers are actively collaborating with industry and research bodies to transfer their expertise to energy storage, semiconductor manufacturing, and even quantum technology development. The continued push for miniaturization, automation, and AI-driven data analysis in plasma diagnostics—exemplified by the integration efforts at ITER Organization—suggests a robust outlook for further cross-sector innovation. As a result, the engineering advances from tokamak diagnostics are set to play a pivotal role in enabling more precise, efficient, and reliable diagnostic solutions across a spectrum of critical industries.
Investment Trends and Funding Opportunities
The field of tokamak plasma diagnostics engineering is witnessing notable growth in investment and funding, propelled by the global pursuit of practical fusion energy. As of 2025, financial support is distributed among public research institutions, government-backed mega-projects, and an emergent cohort of private fusion startups. The European Union’s ongoing commitment to the Fusion for Energy (F4E) program, which funds the ITER project, ensures a stable base for diagnostic R&D, supporting contracts with diagnostics system integrators and specialist suppliers. ITER’s diagnostics procurement, valued at over €1 billion, is expected to sustain opportunities through 2030 as systems transition from design to commissioning.
National programs remain instrumental. The United Kingdom’s UK Atomic Energy Authority (UKAEA) continues to channel government investment into advanced plasma diagnostics through its STEP and MAST-U projects, with new funding rounds anticipated in the next two years to develop real-time monitoring and machine learning tools. In the United States, the Department of Energy (DOE) maintains competitive grant mechanisms for universities and industry partners via the Fusion Energy Sciences program to advance diagnostic instrumentation for the DIII-D and NSTX-U tokamaks, with a 2025–2027 budget expected to surpass $600 million for fusion research infrastructure.
Private sector interest is accelerating, as exemplified by recent investments in firms like Tokamak Energy and TAE Technologies, both of which are scaling up diagnostic engineering capabilities for prototype reactors. These companies have secured funding rounds exceeding $100 million each since 2022, with further capital injections targeting the integration of advanced sensors and data platforms for plasma behavior analysis. Strategic partnerships with diagnostics specialists—such as D-TACQ Solutions (data acquisition) and HEDTKE Ingenieurbüro (plasma impurity monitors)—are anticipated to multiply as commercial demonstration reactors move toward construction.
Looking ahead, funding opportunities are expected to expand through public-private partnerships, with a focus on scalable diagnostics that enable autonomous control, real-time safety monitoring, and predictive plasma modeling. The proliferation of fusion pilot plants by the late 2020s should further stimulate investment in diagnostic engineering, with procurement forecasts indicating rising demand for robust, cost-effective systems across the sector.
Future Outlook: Roadmap to Commercial Fusion Energy
Tokamak plasma diagnostics engineering is poised for significant advancements in 2025 and the following years, as international fusion projects transition from experimental phases to large-scale demonstration and pre-commercial operations. The central role of diagnostics in achieving reliable, high-performance plasma regimes and ensuring operational safety is widely recognized, driving both technological innovation and global collaboration.
A key milestone in 2025 will be the continued integration and commissioning of advanced diagnostic systems within the ITER Organization tokamak. ITER’s diagnostics suite, comprising over 50 distinct systems, sets a new benchmark for complexity and scale. These include Thomson scattering, neutron flux monitors, bolometers, and real-time plasma position sensors, each engineered to withstand high neutron and gamma fluxes, tritium environments, and intense electromagnetic interference. As ITER ramps up its first plasma operations, the efficacy and reliability of these diagnostics will shape the operational parameters and inform design choices for future commercial reactors.
Simultaneously, leading private-sector fusion ventures such as Tokamak Energy and TAE Technologies are investing in compact, modular diagnostics tailored for rapid prototyping and real-time machine learning analysis. These companies focus on robust, miniaturized sensor arrays, fast imaging systems, and advanced data acquisition hardware to facilitate agile development cycles and optimize plasma confinement and stability. Their engineering solutions are likely to influence the broader industry, emphasizing scalability, reliability, and cost-effectiveness for future commercial fusion plants.
Materials and component suppliers, including Oxford Instruments and Mirion Technologies, are developing radiation-hardened detectors and high-precision optical systems to meet the escalating demands of next-generation tokamaks. These advances aim to improve diagnostic longevity, accuracy, and maintainability, especially as devices move toward longer pulse durations and higher fusion power outputs.
On the regulatory and standardization front, organizations such as the International Atomic Energy Agency (IAEA) are leading efforts to harmonize diagnostic performance standards and data sharing protocols. In 2025 and beyond, increased international collaboration is anticipated to accelerate the development of open-source diagnostic software and reference databases, fostering interoperability and expediting the path to commercial fusion energy.
Overall, tokamak plasma diagnostics engineering in 2025 is characterized by a robust convergence of public and private innovation, material science progress, and regulatory coordination—each critical for the realization of reliable, economically viable fusion power in the coming decade.
Sources & References
- ITER Organization
- EUROfusion
- Thales
- Mirion Technologies
- Tokamak Energy
- TAE Technologies
- D-TACQ Solutions Ltd
- American Superconductor Corporation
- Japan Atomic Energy Agency
- General Atomics
- AMETEK
- Commonwealth Fusion Systems
- Helion Energy
- Kyocera
- Thorlabs
- Canon
- Hamamatsu Photonics
- TOPTICA Photonics
- Radiometer Physics GmbH
- Fusion for Energy
- Thermo Fisher Scientific
- Oxford Instruments
- International Atomic Energy Agency (IAEA)
- UK Atomic Energy Authority