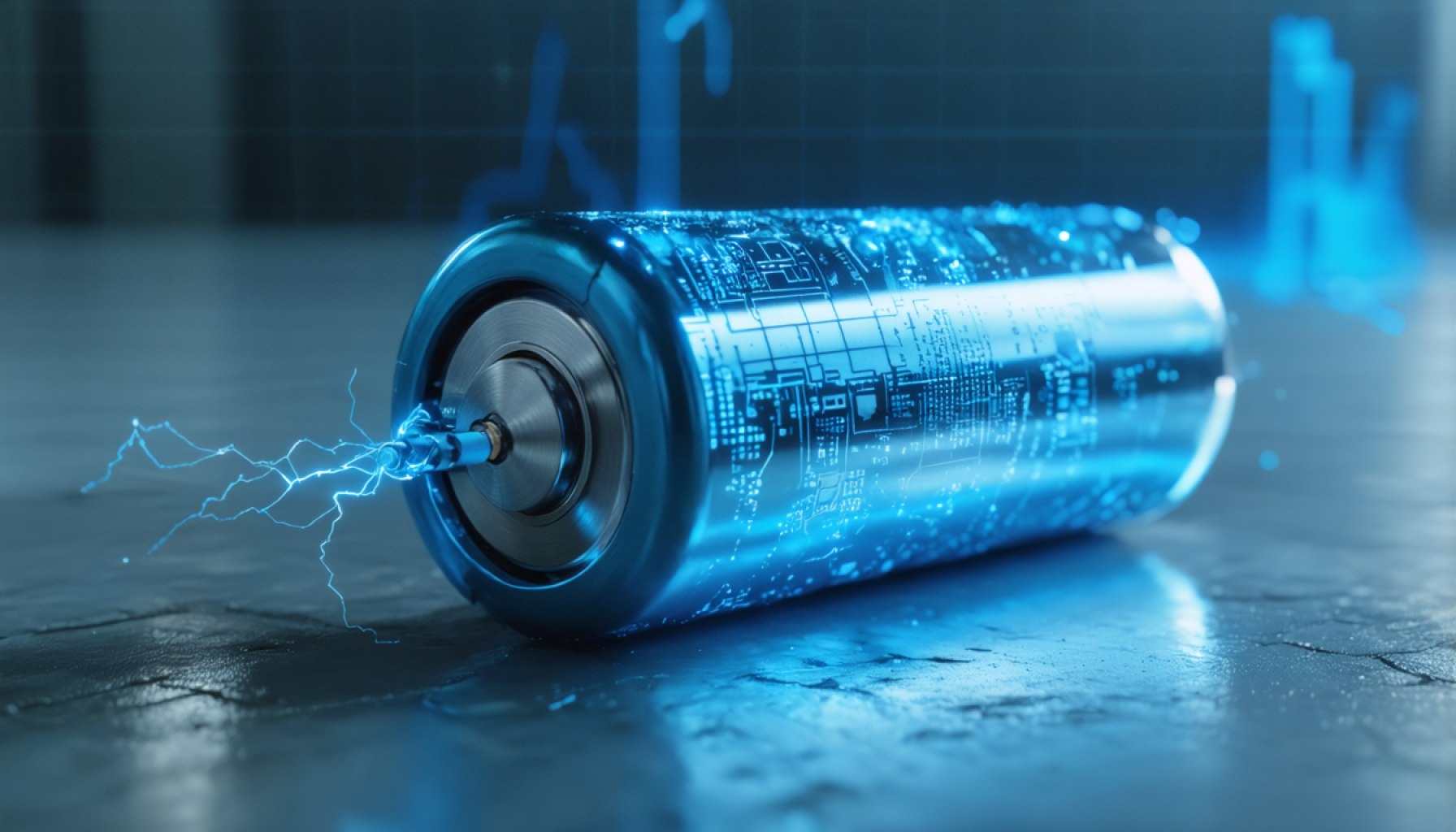
- Solid-state lithium metal batteries (SSBs) suffer from early failures due to metal fatigue rather than unstable chemical reactions.
- Lithium metal anodes experience stress from expansion and contraction during charge cycles, leading to microcracks and decreasing battery lifespan.
- Microcracks at the anode-electrolyte interface foster the growth of lithium dendrites, which can cause short circuits.
- The Coffin-Manson law, typically used to predict metal fatigue, is applicable to understanding SSB wear patterns.
- This research offers insights into enhancing battery cycle life by mitigating mechanical stress, promising improved energy storage for electric vehicles and devices.
- The study highlights the critical role of integrating mechanics with chemistry to advance sustainable energy technologies.
Imagine bending a paperclip repeatedly. With each twist, tiny cracks appear, weakening its structure until, finally, it snaps. Such is the fate of promising solid-state lithium metal batteries (SSBs), a cutting-edge technology that could revolutionize energy storage but often falls short due to a surprisingly familiar culprit: metal fatigue.
New research reveals that the root of these early failures is not due to unstable chemical reactions, as many believed, but rather mechanical stressors akin to those in the humble paperclip. At the heart of this issue lies the lithium metal anode, a key component meant to deliver high energy density. Yet, as it expands and contracts with each charge cycle, it undergoes repeated stress that leads to microscopic fractures—prematurely shortening the battery’s lifespan.
Researchers, led by Tengrui Wang, have uncovered through detailed scanning electron microscopy and sophisticated simulations, that these microcracks at the anode-electrolyte interface are the primary breeding ground for troublesome lithium dendrites. These needle-like structures pose a lethal threat to battery integrity, sometimes piercing through the solid electrolyte to trigger short circuits.
What makes these findings intriguing is the application of mechanical laws, particularly the Coffin-Manson law, which traditionally predicts fatigue failures in metals. This revelation suggests that like bridges and other critical structures, lithium metal anodes in SSBs wear and tear follows predictable patterns. Leveraging this knowledge could pave the way for engineers to fortify these batteries against metal fatigue.
For those who dream of electric vehicles with extended range and gadgets that charge in a flash, the understanding of lithium’s fatigue offers a beacon of hope. Researchers now have a roadmap to enhance the cycle life of these batteries, focusing on innovative solutions that mitigate mechanical stress, ensuring that the promise of safer, longer-lasting energy storage is within reach.
Ultimately, this breakthrough underscores a vital lesson: the interplay of mechanics and chemistry is paramount in unlocking the full potential of advanced technologies. Addressing these nuances could mean the difference between a breakthrough and a breakdown in the race for sustainable energy.
Unlocking the Full Potential of Solid-State Lithium Metal Batteries: Beyond Metal Fatigue
Introduction
In the quest for revolutionary energy storage, solid-state lithium metal batteries (SSBs) have emerged as a promising solution. However, akin to a paperclip bending under stress, these batteries often suffer from mechanical failures due to metal fatigue. Recent research, led by Tengrui Wang, sheds light on the true nature of these failures, offering a comprehensive understanding that could lead to improved battery technology.
Key Facts and Emerging Insights
1. Metal Fatigue in SSBs:
– The central challenge in SSBs is the lithium metal anode, which experiences mechanical stress during charge and discharge cycles. These stresses cause microcracks and eventually lead to the growth of lithium dendrites.
– Unlike traditional misconceptions focused on chemical instabilities, it is the mechanical properties that play a crucial role in battery degradation.
2. Applying the Coffin-Manson Law:
– Traditionally used in predicting metal fatigue in infrastructure like bridges, the Coffin-Manson law now helps explain fatigue patterns in lithium metal anodes.
– Engineers can use this law to predict the lifespan of SSBs and design more robust structures to prevent premature failure.
3. Technology Solutions:
– Incorporating flexible materials or coating technologies at the anode-electrolyte interface can mitigate mechanical stress.
– Advanced 3D architectures could also distribute strain more evenly, potentially reducing the risk of crack formation.
4. Impacts on Energy Storage:
– SSBs promise to drastically improve the range of electric vehicles and the efficiency of electronic devices through faster charging times and longer battery life.
– Addressing mechanical fatigue could extend battery life significantly, ensuring more reliable and sustainable energy solutions.
Market Forecast and Industry Trends
– Growing Demand: With a surge in electric vehicle adoption and the need for high-performance consumer electronics, the demand for reliable SSBs is projected to increase exponentially.
– Research and Investment: The battery industry is witnessing large investments in research to overcome mechanical challenges. Companies are focusing on both scalability and sustainability to cater to emerging markets.
Pros and Cons Overview
Pros:
– Higher energy density compared to traditional lithium-ion batteries.
– Potential for longer cycle life when mechanical issues are addressed.
– Enhanced safety with reduced risk of thermal runaway.
Cons:
– Current limitations in architecture and material costs.
– Mechanical stress leading to microcracks remains a pressing challenge.
– Dendrite formation could pose safety risks unless adequately managed.
Actionable Recommendations
– Innovative Material Use: Focus on developing composite materials that can withstand mechanical variations during battery operation.
– Design Optimization: Implement designs that account for mechanical laws and stress distribution methods to lessen crack propagation.
– Increased Collaboration: Cross-disciplinary approaches involving material science, mechanical engineering, and chemistry will accelerate breakthroughs.
Conclusion
Understanding and addressing the mechanical components of lithium metal anodes is crucial for unlocking the potential of SSBs. As researchers and engineers develop solutions to enhance their durability, the promise of cutting-edge battery technology becomes within reach. For companies and consumers alike, these innovations could herald a new era of efficient, reliable, and sustainable energy solutions.
For further reading on the wider context of energy storage advancements, check out the latest updates at Popular Mechanics and stay informed with expert opinions and emerging trends.
Closing Thought
Those interested in exploring battery technology further should keep abreast of advancements in materials science and mechanical engineering practices. By doing so, you can gain insights into the future landscape of energy storage and its applications across industries.